Editor’s note: David Clemens-Sewall, a research physical scientist at NOAA’s Physical Sciences Laboratory, provided the following blog post.
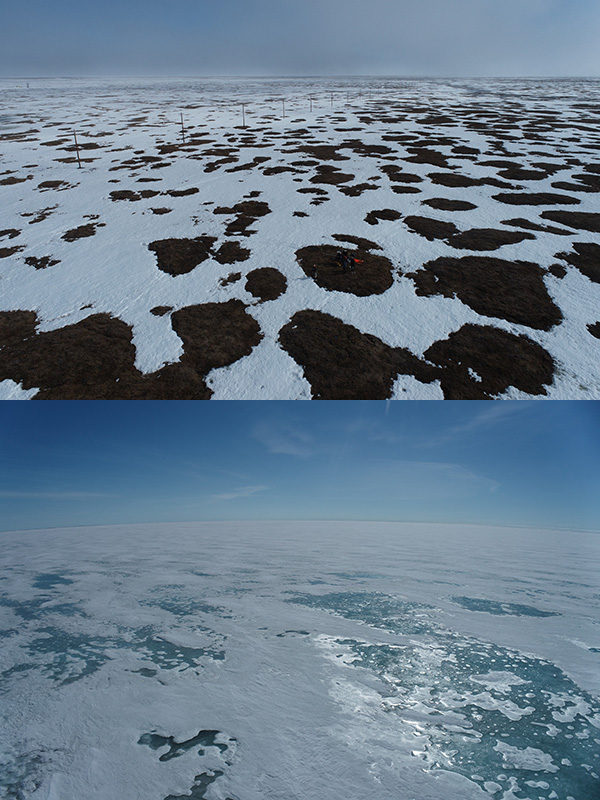
Every spring in the Arctic, tundra and sea ice undergo a striking transition: The cold, white, reflective snow cover melts away to reveal darker land, sea ice, and—after the ice melts—ocean surfaces. Along the way, liquid water released from snowmelt drives changes to the surface that further accelerate snow and ice melt: first, by darkening the snow by changing the character of the snow grains—snow metamorphism—and then by forming relatively dark puddles of meltwater—or melt ponds—on the sea ice and tundra. The critical scientific parameter that quantifies this darkening, which drives the melt, is albedo: the proportion of incoming sunlight reflected away by the surface.
Cold, dry, snow-covered surfaces can have albedos greater than 0.8 (more than 80% of incoming sunlight is reflected). Dark tundra, melt ponds, and open ocean can have albedos less than 0.2 (more than 80% of the incoming sunlight is absorbed, warming the surface). The cycle of snow and ice melt decreasing albedo and causing faster melt is known as the snow or sea ice albedo feedback, and it is a major reason why the Arctic is warming faster than the global average.
Despite decades of improvements, many models still do not accurately simulate the observed albedo feedback (e.g., the Community Model Intercomparison Project version 6; Thackeray et al. 2021). The Snow ALbedo eVOlution (SALVO) field campaign, supported by the U.S. Department of Energy’s ARM user facility and Atmospheric System Research (ASR) program area, was devoted to measuring snow and albedo in Utqiaġvik, Alaska, to help address this weakness in models.
The springtime transition from snow-covered to snow-free surfaces does not proceed in lockstep synchrony on spatial scales from meters to continents. Even on scales of just hundreds of meters, SALVO observed that some areas “melt out” weeks before other areas on sea ice and tundra (Pinzner et al. 2024; also Figure 1). Studies using earth system models have found that snow albedo changes as small as 0.02 can have big impacts on the Arctic, such as changing the projected onset of seasonally sea ice-free conditions by decades (e.g., Kay et al. 2022). A critical challenge for our SALVO team was: How do we accurately and efficiently measure albedo in this spatially heterogeneous environment?
Minimizing Points for Measurement Error
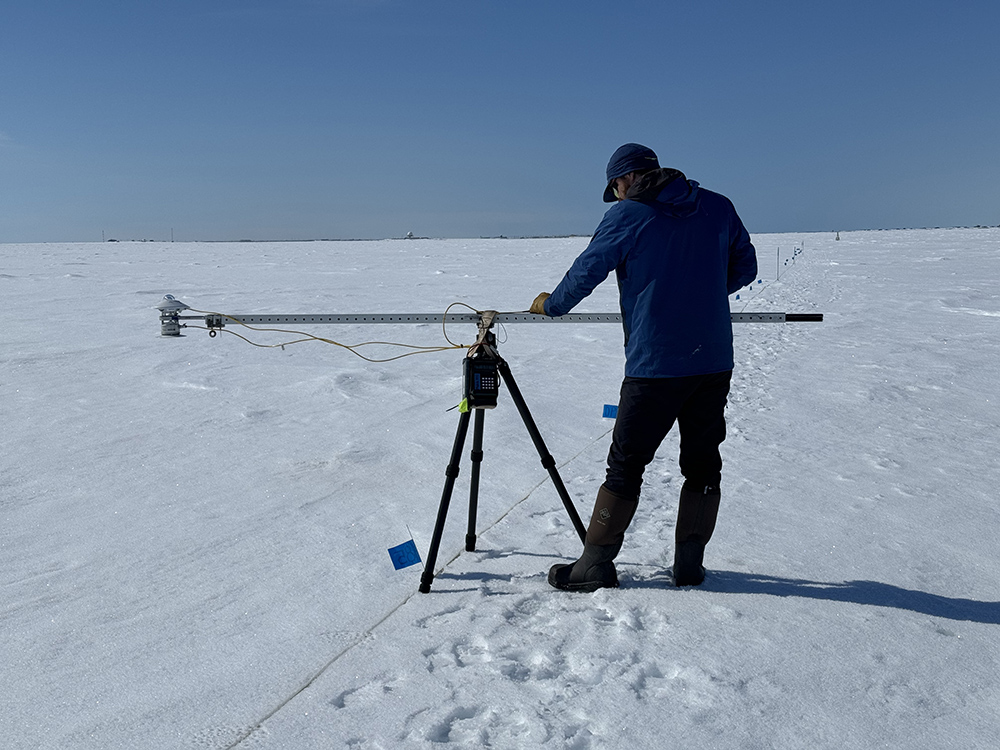
Broadband (full-solar spectrum) albedo measurements are typically made with paired upward- and downward-looking pyranometers—insulated instruments that produce a voltage proportional to the amount of sunlight received by the sensor. At fixed sites such as ARM’s North Slope of Alaska (NSA) atmospheric observatory, which hosted SALVO, the upward-looking pyranometers are often integrated with tilt sensors and sun trackers that allow independent measurements of the direct solar beam and diffuse sunlight. The downward-looking pyranometers are elevated several meters above the ground with the intention of observing a “representative” footprint.
However, for spatially distributed measurements, SALVO mounted pyranometers on a boom attached to a tripod (Figure 2), which we carried to each measurement site. The boom setup was designed and built for SALVO in collaboration with the University of Alaska Fairbanks machine shop.
For field measurements of broadband albedo, there are two primary sources of error. First, the instrument operator and the tripod legs are within the field of view of the downward-looking sensor, so some effect on the measurements is inevitable. To reduce the impacts to an acceptable level, the sensor is placed at the end of the boom, which is longer than the sensor’s height above the surface. Because the operator must carry the instrument, this practically limits the sensor’s height to ~1 meter above the surface. In addition, the tripod must be approximately balanced to hold the instrument steady for a measurement. So, we extended the boom on the operator side of the tripod and placed a counterweight at its end. The longer the boom extension, the less total weight is needed to balance the system—an important consideration when you are carrying the instrument!
The second major source of error for field albedo measurements is imprecise leveling of the upward-looking pyranometer. If the sensor is tilted just a little bit toward the sun, it will overestimate the true amount of energy coming in from sunlight, and vice versa if tilted away from the sun. The sensor comes with a built-in bubble level for this reason, but our field setup complicates leveling the sensor. The bubble level is out at the end of a boom 1.5 meters away from the operator, who cannot read it from where they are positioned. To address this, we mounted a second bubble level on the operator side of the boom, which we aligned with the level on the sensor before each set of measurements.
To collect a measurement, the operator would first position the tripod at the measurement site, then hold the boom level for ~10 seconds (double the integration time of the sensor), and take a measurement by pressing the button on the data logger. With this setup, an operator could measure an entire 200-meter albedo line at a spacing of 5 meters (41 total broadband albedo measurements) in ~30 minutes.
What the Data Show
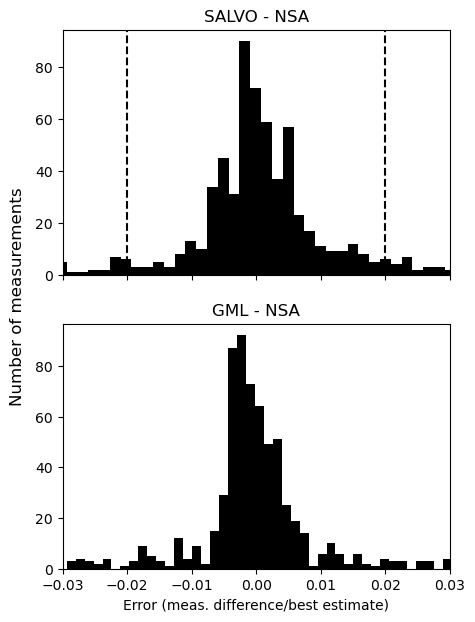
The primary SALVO measurement site was within ~200 meters of the NSA’s pyranometers. We assessed the uncertainty of SALVO’s observations using measurements of incoming sunlight at the NSA and the adjacent NOAA Global Monitoring Laboratory (GML) Barrow Atmospheric Baseline Observatory (Figure 3). For albedo, the relevant metric is the error in the incoming sunlight as a proportion of the total amount of sunlight. The median absolute deviation (a robust measure of distribution spread) for the difference between the SALVO and NSA incoming measurements was 0.005. Furthermore, by comparing the incoming measurements made at the GML and NSA, which are also ~200 meters apart, we found that about 40% of the difference between the SALVO and NSA measurements could be explained by real differences in the incoming sunlight at the two locations. Note that our target for the total error in albedo was less than 0.02.
After further processing and quality control, these data will be freely available from the ARM Data Center.
References:
Kay J, P DeRepentigny, M Holland, D Bailey, A DuVivier, E Blanchard‐Wrigglesworth, C Deser, A Jahn, H Singh, M Smith, M Webster, J Edwards, S-S Lee, K Rodgers, and N Rosenbloom. 2022. “Less Surface Sea Ice Melt in the CESM2 Improves Arctic Sea Ice Simulation With Minimal Non-Polar Climate Impacts.” Journal of Advances in Modeling Earth Systems, 14(4), 10.1029/2021MS002679.
Pinzner A, M Sturm, J Delamere, and A Mahoney. 2024. “An Examination of Water‐Related Melt Processes in Arctic Snow on Tundra and Sea‐Ice.” Water Resources Research, 60(1), 10.1029/2022WR033440.
Thackeray C, A Hall, M Zelinka, and C Fletcher. 2021. “Assessing Prior Emergent Constraints on Surface Albedo Feedback in CMIP6.” Journal of Climate, 34(10), 10.1175/JCLI-D-20-0703.1.
SALVO at AGU: The SALVO team will present a poster, “How Snow Drives the Springtime Evolution of Surface Albedos in the Arctic Coastal Environment,” at the 2024 American Geophysical Union (AGU) Annual Meeting in Washington, D.C. It will be presented from 8:30 a.m. to 12:20 p.m. Eastern on Tuesday, December 10, in Hall B-C (Poster Hall) at the Walter E. Washington Convention Center.