Three representative ARM small field campaigns investigate aerosol formation, ship exhaust, and evolving albedo
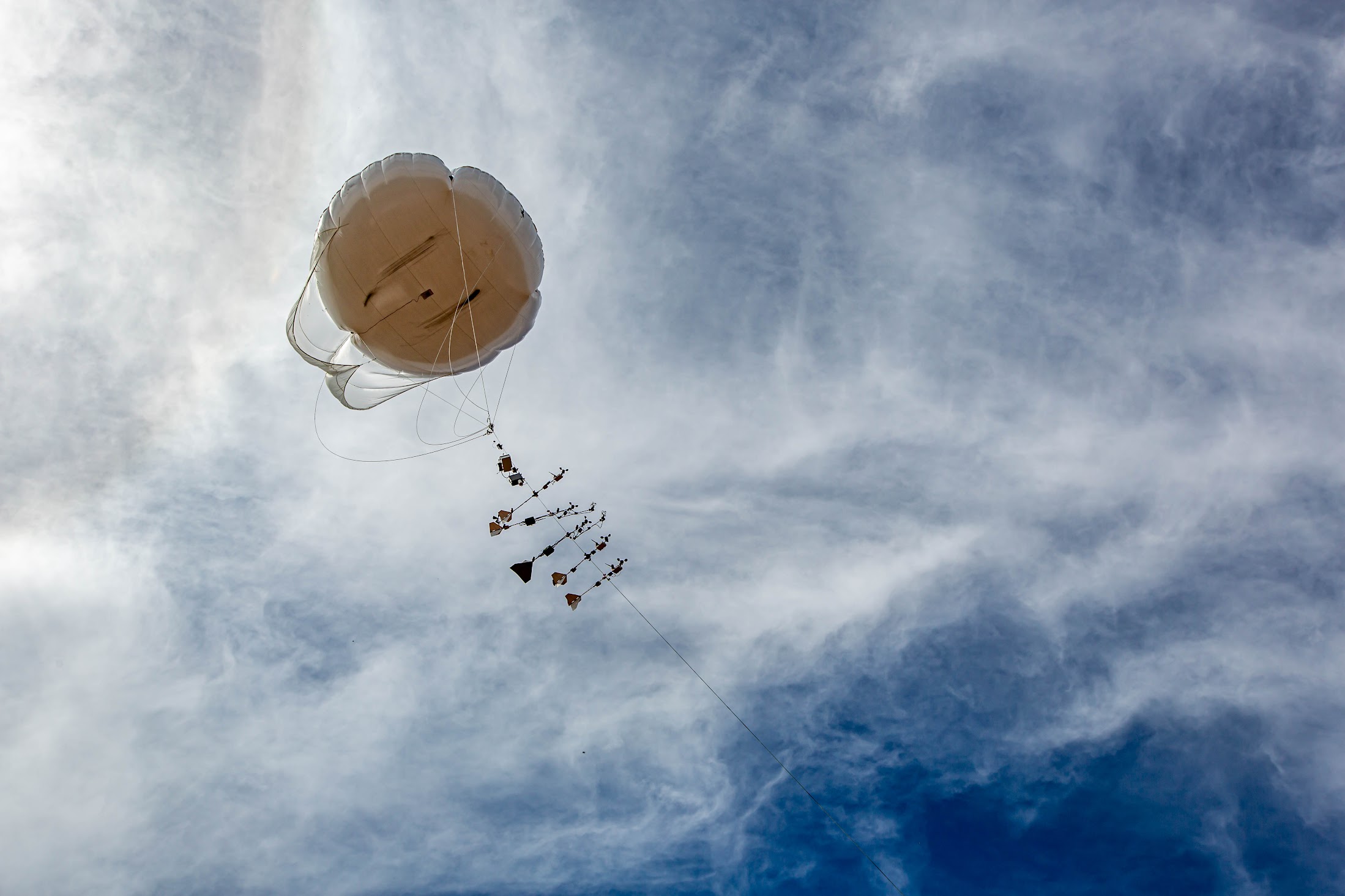
Compared to their larger counterparts in atmospheric research, small field campaigns involve fewer people, smaller budgets, and tighter schedules. However, these time- and money-constrained efforts still take on big scientific questions.
Small campaigns are regularly supported by the U.S. Department of Energy’s (DOE’s) Atmospheric Radiation Measurement (ARM) user facility. ARM operates three fixed and three mobile atmospheric observatories in climate-critical regions of the world.
By ARM’s definition, small campaigns cost no more than $300,000. Funding covers only the use of ARM facilities, instruments, and expertise, not the research itself.
Mike Ritsche at Argonne National Laboratory in Illinois refers to small campaigns as “short-term campaigns” because they typically require just weeks or a few months to complete. (However, ARM does have several multiple yearlong small campaigns.) Ritsche is a member of ARM’s Infrastructure Management Board (IMB), which reviews small-campaign proposals from scientists worldwide. ARM accepts proposals for small campaigns throughout the year.
What ‘Small’ Means
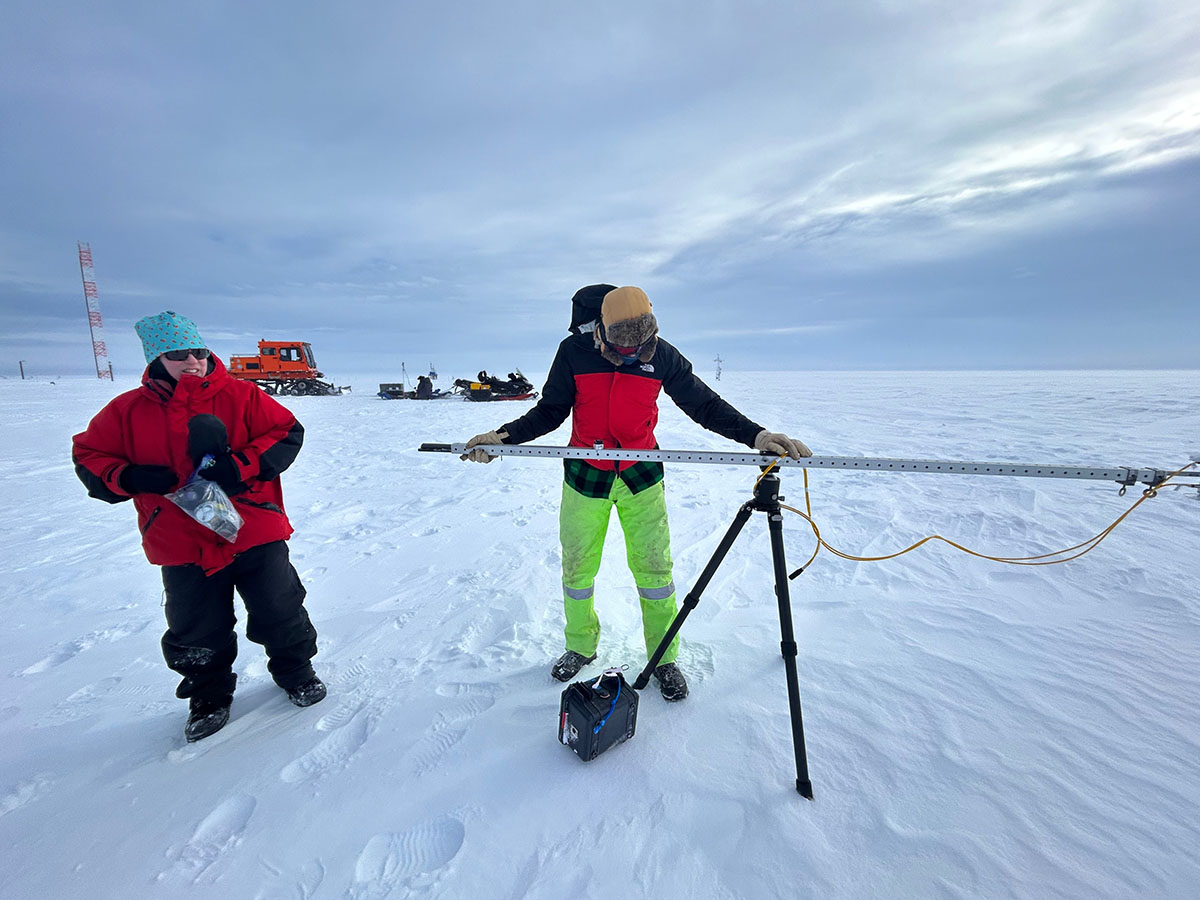
In addition to their short-term nature, ARM small campaigns often:
- generate data complementary to ARM’s instruments
- involve early career researchers
- require only a few people, unlike typical large-scale ARM campaigns
- field-test instruments still under development.
Over the years, ARM has supported deployments of guest instruments developed by both academic researchers and by small businesses funded by DOE’s Small Business Innovation Research program.
Small campaigns are popular among new and veteran ARM users, says Ritsche.
Since 2014, ARM has conducted 314 campaigns, according to the ARM Data Center at Oak Ridge National Laboratory in Tennessee. Most are small.
In fiscal year 2024, the IMB approved 25 small campaigns for ARM’s three fixed-location observatories: 19 in Oklahoma, four in Alaska, and two in the Azores.
Here’s a look at three of those campaigns.
The Chemistry of New Particle Formation
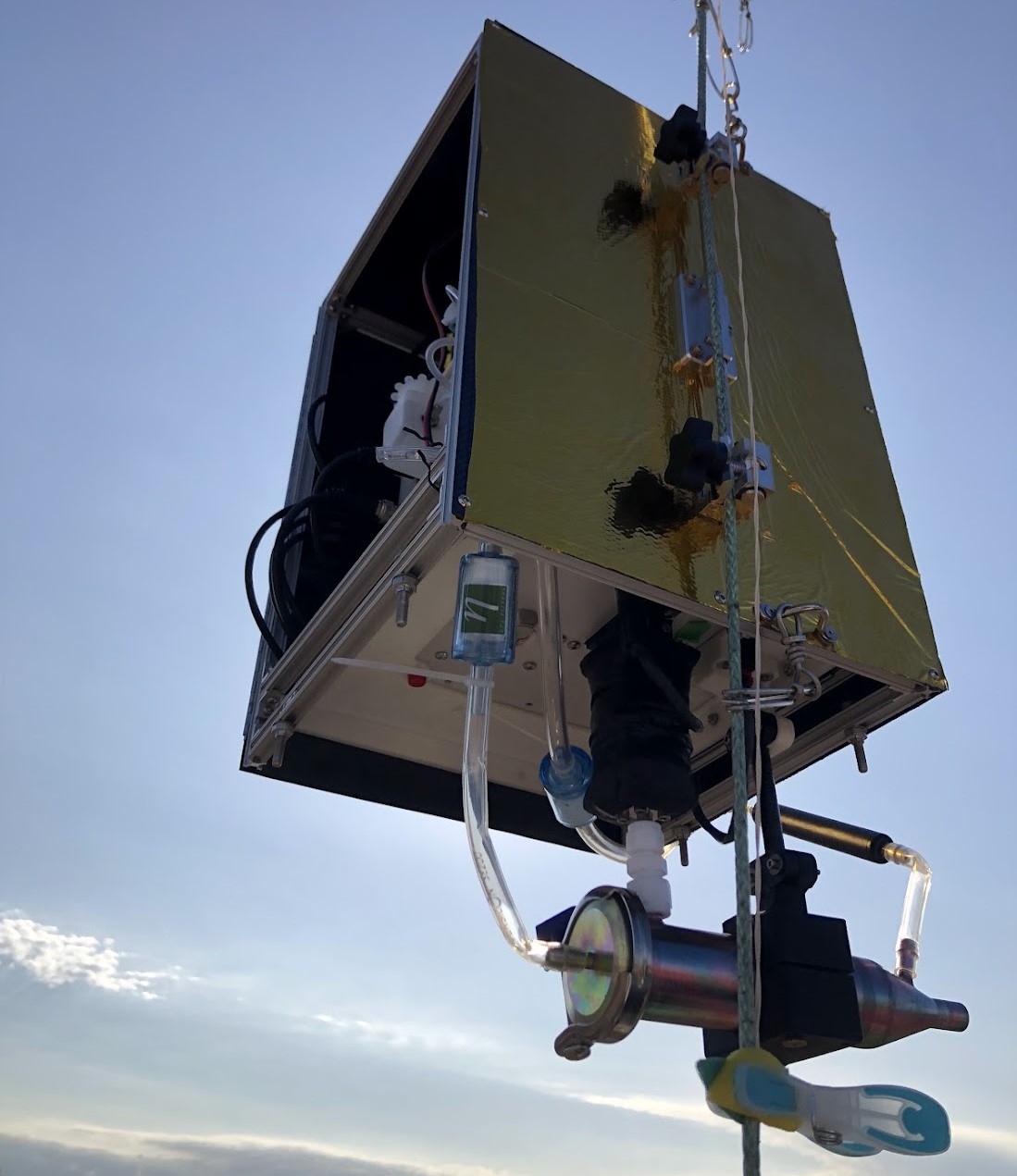
In May and June 2024, Coty Jen, an associate professor of chemical engineering at Carnegie Mellon University in Pennsylvania, used surface and balloon-mounted instruments at ARM’s Southern Great Plains (SGP) atmospheric observatory in Oklahoma to measure gases that lead to new particle formation.
The chemical and physical mechanisms of how new particles become aerosols remain an uncertainty in models that simulate a changing climate. Aerosols are tiny atmospheric particles—hybrids of gases, solids, and water—that make clouds and precipitation possible.
During a small campaign called Vertically Resolved Nucleation Precursors at SGP (VRNPS), Jen and three Carnegie Mellon PhD students deployed a prototype reactive condensation particle counter on ARM’s tethered balloon platform. About the size of a lunchbox, the counter measures concentrations of sulfuric acid that “nucleate,” or initiate, cloud-forming particles.
The SGP’s Guest Instrument Facility also housed Jen’s larger project instruments. They measured the concentration and size of atmospheric aerosol particles and characterized gas chemistry. The group’s main measurement targets were sulfuric acid, related precursor gases, and newly formed particles.
Precursor gases react to form seed-like particles for making cloud droplets. But they start out very tiny—some “as small as two molecules huddled together,” says Jen, whose work was funded by DOE’s Atmospheric System Research (ASR) program.
Tiny but mighty: Worldwide, atmospheric nucleation generates 50% of the seed particles that help form cloud droplets.
Such nucleation begins with gases, primarily sulfuric acid, which initiate particles in ways Jen is trying to observe, measure, and model. One challenge is that sulfuric acid combines with many other compounds. That results in dramatically different particle formation rates, which vary with changes in region and altitude.
Another challenge is that nucleation reactions take place at very low concentrations, sometimes only measurable in parts per quadrillion.
Still, tethered balloons were an ideal platform for Jen’s science mission. They have controlled rates of ascent and can pause at given altitudes. Their operational range, up to a kilometer (about 3,280 feet), is high enough in the atmosphere’s boundary layer to investigate how the vertical distribution of precursor nucleation gases changes with meteorological conditions.
Tethered balloons restrict the size and weight of instruments. But that fit nicely with Jen’s goal of developing a small research-grade device that makes it affordable to measure atmospheric sulfuric acid in the field. Her prototype cost about $20,000, a fraction of the cost of larger, more complex laboratory instruments.
Jen’s team also used the SGP opportunity to test a nucleation potential model. It’s designed to predict nucleation rates even in chemically complex atmospheric systems across the world.
Tracking Ship Tracks
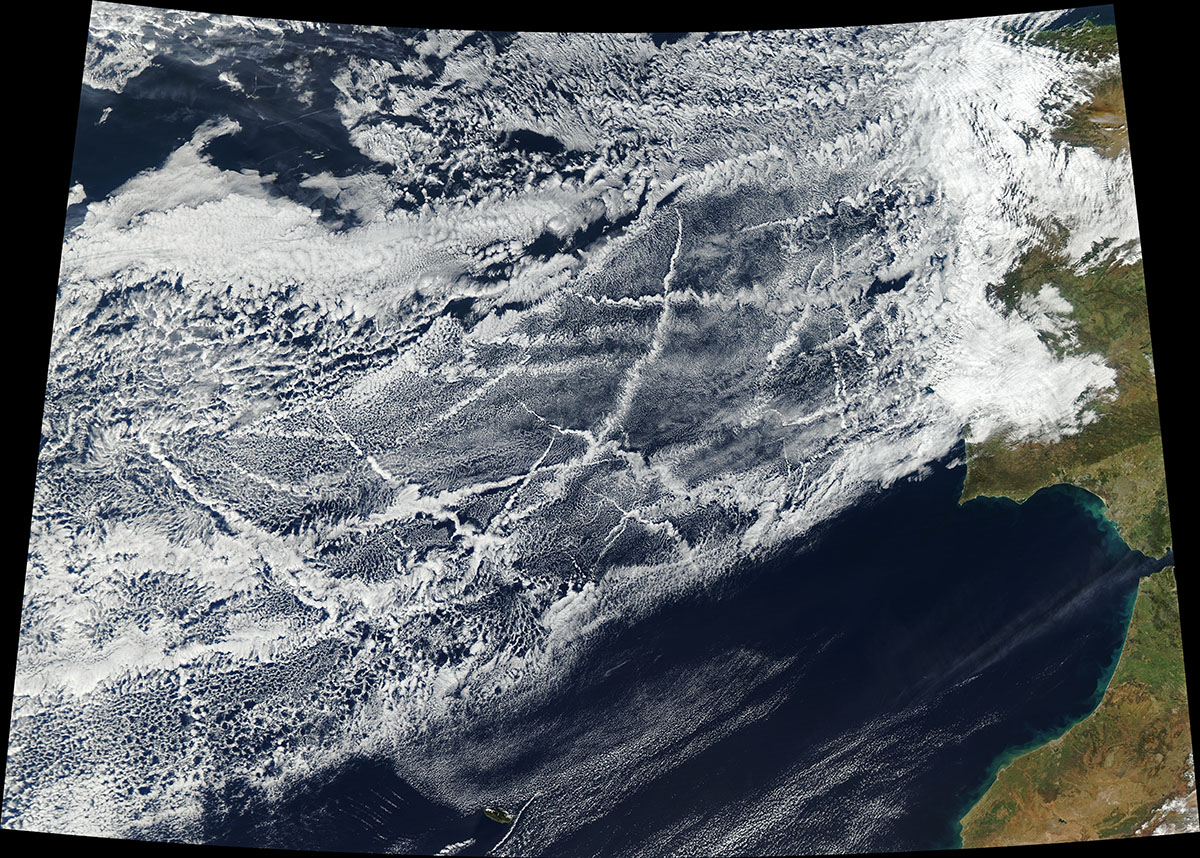
Historically, ships zigzagging across the world’s oceans have burned dirty, high-sulfur diesel fuel. The resulting emissions of sulfur, other gases, and carbonaceous aerosols created a lot of air pollution in the open ocean. But the same emissions also generated cloud-like ship tracks whose brightness and reflectivity had a global cooling effect.
In 2020, the U.N. International Maritime Organization implemented new global fuel standards to address (or help reduce) sulfur pollution in the open ocean.
Since then, scientists have been studying how these regulations are changing the levels of sulfur in the atmosphere; how those changes affect marine clouds and their radiative properties; and how changes in shipping emissions are linked to observed increases in temperatures over the ocean.
Among them was a pair of British scientists that tapped DOE resources.
Plymouth Marine Laboratory chemical oceanographer Mingxi Yang and University of Manchester atmospheric composition professor Hugh Coe led a small ARM field campaign called Impact of Changing Ship Emissions (ACRUISE) that began in 2019 and wrapped up in mid-October 2023.
They collected data from ARM’s Eastern North Atlantic (ENA) atmospheric observatory in the Azores as part of a wider European-American inquiry into the impacts of new regulations on the sulfur content of ship fuels.
ACRUISE is also the acronym for Atmospheric Composition and Radiative forcing changes due to UN International Ship Emissions regulations, a 2019–2024 project funded by the U.K.’s Natural Environment Research Council (NERC). Yang and Coe have related but separate NERC funding awards.
“The ENA (Eastern North Atlantic) site provided a very useful platform. We also benefited from the well-established cloud and radiation measurements.”
“The ENA site provided a very useful platform,” wrote Yang in an email, in part because its remoteness makes it a handy place to measure the evolution of atmospheric sulfur levels in the open ocean. “We also benefited from the well-established cloud and radiation measurements.”
Starting in March 2019, “with wonderful support from the ENA team,” wrote Yang, the ARM portion of ACRUISE installed a high-volume aerosol sampler to collect 24-hour samples once a week. Local staff replaced filters and air-mailed frozen filters periodically to England for analysis of ions, sulfur isotopes, and changes in total sulfate concentrations, post-regulation.
Because of pandemic-related interruptions and changes in personnel, chemical analyses were “substantially delayed but are now underway,” he added.
Data from the ARM fraction of ACRUISE—destined to be included in a future paper, wrote Yang—will be combined with measurement sources from the larger, NERC-funded project. Those include surface sites, research aircraft, and satellites.
Also ahead, he added, are large-scale and high-resolution ACRUISE-informed models of aerosol-cloud interactions and the impact of ship emissions on clouds and their radiative properties.
More broadly, Yang sees ACRUISE as one element in unraveling “highly complex and nonlinear” aerosol-cloud interactions, he wrote, which are “the largest uncertainty in our understanding of the Earth’s radiative balance and in any future climate projections.”
SALVO 2024: Chasing the Melt
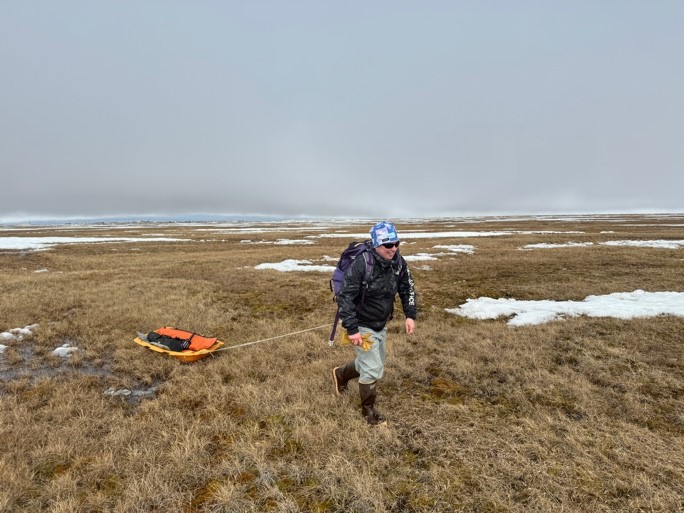
Jennifer “Jen” Delamere, an associate research professor at the University of Alaska Fairbanks, recently wrapped up her lead role in the final phases of a small ARM campaign called Snow ALbedo eVOlution (SALVO). This ARM- and ASR-supported mission started in Alaska in 2019 to document the snow-to-no-snow springtime transition.
During this transition, she says, very bright surfaces no longer reflect 80% of sunlight back into the atmosphere. Instead, they become predominantly dark surfaces that absorb 80% of sunlight, which warms soils, water, and vegetation.
This springtime transition turns “the arctic world from white to dark,” says Delamere. “You can go from this very white surface to—boom!—tundra within a week.”
In April, May, and June 2024, near ARM’s North Slope of Alaska (NSA) atmospheric observatory, Delamere led the final measurement stages of SALVO.
The term “albedo” (derived from the Latin word for “white”) describes the reflectivity of Earth’s surface. Changes in albedo are amplified in the Arctic, but they affect warming trends on a global scale.
The first SALVO leg, led by Delamere’s university colleague Matthew Sturm, produced one springtime investigation of evolving albedo in 2019. Delamere served as a co-investigator on that leg before leading the final two legs of SALVO, the first in 2022 and the second in 2024. Sturm was a co-investigator on the last two legs.
For 10 days in April 2024, while there was still snow cover, members of Delamere’s team were out in the field to collect benchmark data. From May 20 through June 20, they measured changing albedo on a variety of surfaces and topography.
Delamere and her 2024 SALVO team—from five to nine people, depending on work requirements—set up three instrumented transects 200 meters by 25 meters (656 feet by 82 feet) in size. Two were on tundra and one on sea ice—complex terrain covered with snow nine months of the year, where land, ocean, and sea ice meet.
To get even more data for a library of representative landscapes, Delamere and her team laid out additional study swaths about 1,500 meters (4,900 feet) long. They measured thousands of snow depths, snapped aerial photographs, and investigated drained lakes, shallow and deep melt ponds on tundra and sea ice, and various types of tundra vegetation.
The team also studied the impacts on albedo of the distinctive polygonal land features that appear when subsurface permafrost in the Arctic is exposed by the elements.
“As the team became faster and faster with measurement sequences, we were able to chase the melt.”
“As the team became faster and faster with measurement sequences, we were able to chase the melt,” says Delamere.
She credits special contributions from the NSA support team based at Sandia National Laboratories in New Mexico, as well as Jimmy and Josh Ivanoff, full-time observers at the NSA who work for Ukpeaġvik Iñupiat Corporation (UIC) Science.
One SALVO paper has appeared so far, in January 2024, based on 2019 and 2022 fieldwork.
In December 2024, the team plans to present a poster on surface albedo evolution at the annual meeting of the American Geophysical Union.
# # #Author: Corydon Ireland, Staff Writer, Pacific Northwest National Laboratory
ARM is a DOE Office of Science user facility operated by nine DOE national laboratories.